Climate change as an adaptive challenge
Knovel just published my latest white paper, titled Climate Change as an Adaptive Challenge (it’s a free download, but you’ll need to type in some info about yourself before downloading). The blog post below summarizes the argument. The white paper itself contains the latest data demonstrating the case for urgent action, and it ties in nicely to the recently released National Climate Assessment.
Introduction
In their new book Moments of Impact, Chris Ertel and Lisa Kay Solomon, citing Ronald Heifetz, describe two types of challenges:
• Technical challenges are those we can solve using well-known techniques and tools. Such problems are well defined and well understood
• Adaptive Challenges, on the other hand, are “messy, open-ended, and ill defined”. The tools needed to address them may not yet exist. Such problems require different kinds of leadership and problem solving skills, and cry out for interactive engagement among all the people needed to solve them.
Ertel and Solomon write
“It’s nearly impossible for any one senior executive–or small leadership team–to solve adaptive challenges alone. They require observations and insights from a wide range of people who see the world and your organization’s problems differently. And they require combining those divergent perspectives in a way that creates new ideas and possibilities that no individual would think up on his or her own.” [1, page 10]
Climate change is the ultimate adaptive challenge, because the rate and scope of the changes needed to solve the problem will stretch us to the limit. In addition, the solutions must involve changes in behavior and institutional structure, not just technology, because the problem is so pressing. As I argued in Cold Cash, Cool Climate,
Climate change is probably the biggest challenge modern humanity has ever faced. It’s bigger than World War II, because it will take decades to vanquish this foe. It’s harder than ozone depletion, whose causes were far less intertwined with industrial civilization than fossil fuels and other sources of greenhouse gases. And it’s more intractable than the Great Depression (or our current economic malaise) because financial crises eventually pass, assuming we learn from past mistakes and fix the financial system (again!).” [2]
We have many options for reducing greenhouse gas emissions, but we’ll need new ones, too. Existing options will only get us so far. That’s why we’ll have to take an evolutionary approach to this problem, one that embraces the adaptive nature of the challenge before us.
As Ertel and Solomon argue, tackling adaptive challenges requires “strategic conversations” that help define the problem and generate innovative ideas for solving it. The first step in creating such a strategic conversation is to understand the challenge before us, and this blog post and the associated research note (LINK) are intended to foster such understanding.
The case for urgent action
The case for concern about rising greenhouse gas (GHG) concentrations is ironclad, and the graphics in the white paper show one compelling way to describe that case. We’re on track for more than two doublings of greenhouse gas concentrations by 2100 when all warming agents are included (see Figure 1). Combined with an expected warming of about 3 Celsius degrees per doubling of GHG concentrations (the climate sensitivity) that implies about a 6 Celsius degree warming commitment on our current path (the 5.5 Celsius degree warming calculated by MIT is lower because it takes many centuries for the climate to equilibrate to fully account for the effects of changes in concentrations).
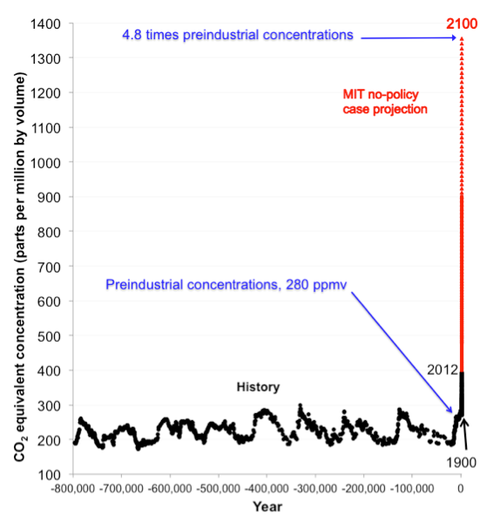
Figure 1: Carbon dioxide equivalent concentrations for the past 800,000 years and projected to 2100 assuming no change in policies, including other warming gases
Sources: CO2 concentration data before year zero taken from a composite record produced by NOAA [ftp://ftp.ncdc.noaa.gov/pub/data/paleo/icecore/antarctica/epica_domec/edc-co2-2008.txt], based on [3, 4, 5, 6, 7]. CO2 concentration data from years zero to 1958 taken from a composite record produced by NOAA [ftp://ftp.ncdc.noaa.gov/pub/data/paleo/icecore/antarctica/law/law2006.txt], based on [8, 9, 10, 11, 12, 13, 14, 15, 16, 17]. Concentrations for years 1959 to 2012 are taken from Keeling et al. [18] and Tans [19]. MIT no-policy case concentrations taken from Sokolov et al. [20]. Negative numbers indicate years BC. Note that y-axis begins at 100 parts per million by volume.
The graphs in my white paper show a dramatic shift in the climate system caused by human activity, one that has no precedent in human history. We need to keep a significant fraction of proved fossil fuel reserves in the ground if we’re to stabilize the climate and avoid these changes. It’s hard to imagine a starker adaptive challenge for humanity, but it’s one that we must confront if we hope to leave a livable world for our descendants.
What can we do?
To meet the climate challenge we’ll need rapid GHG emission reductions in the next few decades. This conclusion is inescapable because it’s cumulative emissions that matter, due to the long lifetime of many greenhouse gases. If we want to prevent global temperatures from increasing more than 2 Celsius degrees, we have a fixed emissions budget over the next century. If we emit more now we’ll have to reduce emissions more rapidly later, so delaying action (either to gather more data or to focus on energy innovation) is foolish and irresponsible. If energy technologies improved as fast as computers there might be an argument for waiting under some circumstances, but they don’t, so it’s a moot point.
Of course, we need new technologies and should therefore invest heavily in research and development, but there are vast opportunities for emission reductions using current technologies, and cost reductions for these technologies are dependent on implementing them on a large scale (learning by doing only happens if we do). So the focus in the next few decades should be on aggressive deployment of current low-emissions technologies, bringing new technologies into the mix as they emerge.
The changes we need are so large that no part of the economy will remain untouched, and that means opportunity. In fact, we’ll probably need to scrap some capital in the energy sector, given the rate of emissions reductions that will be required to maintain a livable climate. Entrepreneurs can lead the way by designing new low-emission products, services, and institutional arrangements that are so much better than what they replace that people are eager to adopt them (and to scrap some of their high emitting existing capital along the way).
Emissions reduction opportunities start by focusing on the tasks we want to accomplish and associating those tasks with flows of energy, emissions, and costs, which you then work to minimize. This focus on tasks frees you from the constraints of how they are currently accomplished and allows you to capture compounding resource savings upstream. By considering the whole system and designing to approach theoretical limits of efficiency, it is often possible to achieve drastically reduced emissions while also improving other characteristics of products or services substantially.
Information and communication technology (ICT) is accelerating the rate of innovation throughout the economy, and that development has implications for business opportunities in this space. ICT speeds up data collection, helps us manage complexity, allows us to restructure our institutions more easily, and lets us rapidly learn and adapt to changing circumstances. It also creates a continuously renewable source of emissions reductions, and is a great place to look for opportunities because it generally offers rapid speed to market and low startup costs.
When considering the climate issue, we can’t avoid the issue of institutional governance. Government has an essential role to play in defining property rights, enforcing contracts, and internalizing external costs. No other institution can do these things, so we need to ensure that these tasks are performed in a way that leads to the kind of society we want. When it comes to government, more is not better. Less is not better. Only better is better. And better is what we as a society should strive for.
Conclusions
Surviving this stage of human development means we’ll need to evolve as a species to learn how to face adaptive challenges like this one. We’ll need to foster rapid innovation, fierce competition, and active coordination between businesses, all at the same time. We’ll also need to change how we think about our responsibilities to each other, to the earth, and to future generations. Innovations in our values can be as powerful as those for new technologies in opening up new possibilities for the future, and these we also need to explore.
The technology now exists for us to move past combustion in most applications, but scaling it up to meet the demands of a modern industrial society won’t be easy. Of course, not doing so will be harder still, because of the damages unrestricted climate change will inflict on the earth and on human society. It’s long past time to get started. There’s simply no more time to waste.
Author’s biography
Jonathan Koomey is a Research Fellow at the Steyer-Taylor Center for Energy Policy and Finance at Stanford University, worked for more than two decades at Lawrence Berkeley National Laboratory, and has been a visiting professor at Stanford University (2003-4 and Fall 2008), Yale University (Fall 2009), and UC Berkeley’s Energy and Resources Group (Fall 2011). He was a lecturer in management at Stanford’s Graduate School of Business in Spring 2013. Dr. Koomey holds M.S. and Ph.D. degrees from the Energy and Resources Group at UC Berkeley, and an A.B. in History of Science from Harvard University. He is the author or coauthor of nine books and more than 200 articles and reports. He’s also one of the leading international experts on the economics of reducing greenhouse gas emissions, the effects of information technology on resource use, and the energy use and economics of data centers. He’s the author of Turning Numbers into Knowledge: Mastering the Art of Problem Solving (which has been translated into Chinese and Italian) and Cold Cash, Cool Climate: Science-Based Advice for Ecological Entrepreneurs (both from Analytics Press).
References
1. Ertel, Chris, and Lisa Kay Solomon. 2014. Moments of Impact: How to Design Strategic Conversations that Accelerate Change. New York, NY: Simon & Schuster.
2. Koomey, Jonathan G. 2012. Cold Cash, Cool Climate: Science-Based Advice for Ecological Entrepreneurs. Burlingame, CA: Analytics Press. [http://www.analyticspress.com/cccc.html]
3. Indermühle, A., E. Monnin, B. Stauffer, T.F. Stocker, and M. Wahlen. 1999. “Atomspheric CO2 concentration from 60 to 20 kyr BP from the Taylor Dome ice core, Antarctica." Geophysical Research Letters. vol. 27, pp. 735-738.
4. Lüthi, D., M. Le Floch, B. Bereiter, T. Blunier, J.-M. Barnola, U. Siegenthaler, D. Raynaud, J. Jouzel, H. Fischer, K. Kawamura, and T.F. Stocker. 2008. "High-resolution carbon dioxide concentration record 650,000-800,000 years before present." Nature. vol. 453, no. 7193. 2008/05/15. pp. 379-382. [http://www.nature.com/nature/journal/v453/n7193/suppinfo/nature06949_S1.html]
5. Monnin, E., A. Indermühle, A. Dällenbach, J. Flückiger, B. Stauffer, T.F. Stocker, D. Raynaud, and J.-M. Barnola. 2001. "Atmospheric CO2 concentrations over the last glacial termination." Science. vol. 291, pp. 112-114.
6. Petit, J.R., J. Jouzel, D. Raynaud, N.I. Barkov, J.-M. Barnola, I. Basile, M. Benders, J. Chappellaz, M. Davis, G. Delayque, M. Delmotte, V.M. Kotlyakov, M. Legrand, V.Y. Lipenkov, C. Lorius, L. Pépin, C. Ritz, E. Saltzman, and M. Stievenard. 1999. "Climate and atmospheric history of the past 420,000 years from the Vostok ice core, Antarctica." Nature. vol. 399, pp. 429-436.
7. Siegenthaler, U., T.F. Stocker, E. Monnin, D. Lüthi, J. Schwander, B. Stauffer, D. Raynaud, J.-M. Barnola, H. Fischer, V. Masson-Delmotte, and J. Jouzel. 2005. "Stable Carbon Cycle-Climate Relationship During the Late Pleistocene." Science. vol. 310, pp. 1313-1317.
8. Etheridge, D.M., L.P. Steele, R.J. Francey, and R.L. Langenfelds. 1998. "Atmospheric methane between 1000 A.D. and present: evidence of anthropogenic emissions and climatic variability." Journal of Geophysical Research. vol. 103, pp. 15979-15996.
9. Etheridge, D.M., L.P. Steele, R.L. Langenfelds, R.J. Francey, J.-M. Barnola, and V.I. Morgan. 1996. "Natural and anthropogenic changes in atmospheric CO2 over the last 1000 years from air in Antarctic ice and firn." Journal of Geophysical Research. vol. 101, pp. 4115-4128.
10. Ferritti, D.F., J.B. Miller, J.W.C. White, D.M. Etheridge, K.R. Lassey, D.C. Lowe, C. MacFarling Meure, M.F. Dreier, C.M. Trudinger, and T.D. van Ommen. 2005. "Unexpected Changes to the Global Methane Budget over the last 2,000 Years." Science. vol. 309, pp. 1714-1717.
11. Langenfelds, R. L., P. J. Fraser, R. J. Francey, L. P. Steele, L. W. Porter, and C. E. Allison. 1996. "The Cape Grim air archive: The first seventeen years, 1978-1995." In Baseline Atmospheric Program Australia. Edited by R. J. Francey, A. L. Dick and N. Derek. Melbourne: Bureau of Meteorology and CSIRO Division of Atmospheric Research. pp. 53-70.
12. Langenfelds, R.L., P.J. Fraser, L.P. Steele, and L.W. Porter. 2004. "Archiving of Cape Grim Air." In Baseline Atmospheric Program Australia. Edited by J. M. Cainey, N. Derek and P. B. Krummel. Melbourne: Bureau of Meteorology and CSIRO Atmospheric Research. pp. 48.
13. Langenfelds, R.L., L.P. Steele, M.V. Van der Schoot, L.N. Cooper, D.A. Spencer, and P.B. Krummel. 2004. "Atmospheric methane, carbon dioxide, hydrogen, carbon monoxide and nitrous oxide from Cape Grimm flask air samples analysed by gas chromatography." In Baseline Atmospheric Program Australia. Edited by J. M. Cainey, N. Derek and P. B. Krummel. Melbourne: Bureau of Meteorology and CSIRO Atmospheric Research. pp. 46-47.
14. MacFarling Meure, C. 2004. The natural and anthropogenic variations of carbon dioxide, methane and nitrous oxide during the Holocene from ice core analysis. Thesis, University of Melbourne.
15. MacFarling Meure, C., D. Etheridge, C. Trudinger, P. Steele,, and T. van Ommen R. Langenfelds, A. Smith, and J. Elkins. 2006. "The Law Dome CO2, CH4 and N2O Ice Core Records Extended to 2000 years BP." Geophysical Research Letters. vol. 33, no. 14. pp. L14810 10.1029/2006GL026152.
16. Sturrock, G. A., D. M. Etheridge, C. M. Trudinger, P. J. Fraser, and A. M. Smith. 2002. "Atmospheric histories of halocarbons from analysis of Antarctic firn air: Major Montreal Protocol species." Journal of Geophysical Research: Atmospheres. vol. 107, no. D24. pp. 4765. [http://dx.doi.org/10.1029/2002JD002548
http://onlinelibrary.wiley.com/doi/10.1029/2002JD002548/abstract]
17. Trudinger, C. M., D. M. Etheridge, P. J. Rayner, I. G. Enting, G. A. Sturrock, and R. L. Langenfelds. 2002. "Reconstructing atmospheric histories from measurements of air composition in firn." Journal of Geophysical Research: Atmospheres. vol. 107, no. D24. pp. 4780. [http://dx.doi.org/10.1029/2002JD002545
http://onlinelibrary.wiley.com/doi/10.1029/2002JD002545/abstract]
18. Keeling, R.F., S.C. Piper, A.F. Bollenbacher, and J.S. Walker. 2009. Atmospheric CO2 records from sites in the SIO air sampling network. Carbon Dioxide Information Analysis Center, Oak Ridge National Laboratory, U.S. Department of Energy, Oak Ridge, Tenn., U.S.A.:
19. Tans, Pieter. 2013. [www.esrl.noaa.gov/gmd/ccgg/trends/]
20. Sokolov, A.P., P.H. Stone, C.E. Forest, R. Prinn, M.C. Sarofim, M. Webster, S. Paltsev, C.A. Schlosser, D. Kicklighter, S. Dutkiewicz, J. Reilly, C. Wang, B. Felzer, J. Melillo, and H.D. Jacoby. 2009. Probabilistic Forecast for 21st Century Climate Based on Uncertainties in Emissions (without Policy) and Climate Parameters. Cambridge, MA: Massachusetts Institute of Technology (MIT) Joint Program on the Science and Policy of Climate Change. 169. January. [http://globalchange.mit.edu/files/document/MITJPSPGC_Rpt169.pdf]